CPT Q. 030: Are the tectonic mechanisms affecting the world’s geology primarily vertical or horizontal?
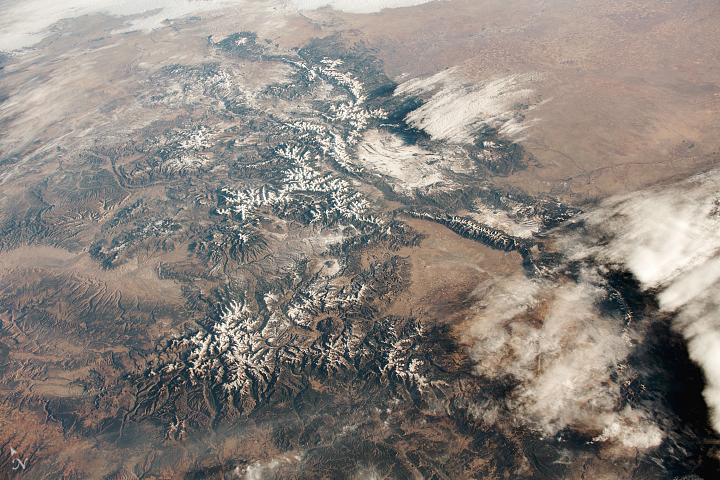
Q. 30. It has been asserted that the “fundamental tectonic mechanisms of global geology are vertical” and that horizontal tectonic mechanisms play only a minor role. Would you please provide your view regarding that assertion?
Response: In regard to the issue of vertical versus horizontal tectonics, let me submit that it is not really a matter of either/or, but that horizontal tectonics and vertical tectonics are intimately tied together. However, when a person excludes the possibility that any significant horizontal tectonic changes could have occurred during the Flood, such a person it seems to me has also excluded most of the available options for explaining the vertical tectonic changes that took place. The two main examples of vertical tectonics cited by the advocate of that view include 1) the uplift of the Rocky Mountains in Wyoming and Montana and 2) the formation of the abyssal hills that cover so much of the deep ocean floor.
Let me first note that the uplift of the young mountain belts of the world—not only of the Rockies, but also of the Andes, the Alps, and the Himalaya—represents spectacular examples of vertical tectonics. So any serious discussion of vertical tectonics must include the mechanics responsible for the uplift of these mountain chains. Amazingly, most of the actual uplift has occurred very recently, much of it during the Pliocene and Pleistocene, corresponding to the very end of the Flood and the centuries immediately following. Moreover, all of these mountain belts today are very near to being in isostatic balance, which implies the considerable mass of rock corresponding to the mountain that lies above nearby ground level is being supported and compensated by a low-density crustal root. For the world’s highest mountains, the roots extend as deep as about 70 km, whereas the typical continental crustal thickness is about 35-40 km. Hence, somehow beneath all these mountain belts there has occurred a considerable increase in the thickness of the continental crust, which has a density some 15% less than the underlying mantle.
When and by what process did this crustal thickening occur? As I will discuss below, almost in all cases it involved horizontal tectonics, specifically, subduction. In some cases, the crustal thickening occurred by lateral transport of lower crust by traction of a horizontally subducting slab below. In many cases it was largely a result of subduction-related volcanism that emplaced significant volumes of low density crustal rock into existing continental crust in a narrow belt not far inland from a subduction zone. In a rare circumstance, one continent subducted beneath another, resulting in a crustal thickness double the normal value.
The fact that the uplift occurred nearly simultaneously for all these mountain chains is a strong argument in favor of the Biblical account of the earth’s physical history and against the uniformitarian account. The time constant for isostatic response is relatively short. In the Biblical framework, the crustal thickening all occurred during the few months of the Flood, and it is logical that most of the isostatic response would be afterward and also be essentially synchronous. By contrast, in the uniformitarian framework, the thickening is stretched out over tens of millions of years, and the isostatic response should have been as well.
Let us first consider the uplift of the Rocky Mountains, which was indeed a spectacular process. I will focus on the Colorado Front Range, since I am personally more familiar with that area, but the same basic mechanics were involved in Wyoming and Montana further to the north. Below are a satellite image and a geological map of part of Colorado showing the abrupt transition between the Great Plains to the east and the Colorado Front Range immediately to the west.
In terms of the Flood, based on geological relationships, this dramatic uplift occurred near the end of the Flood to a few years afterward. But what could possibly cause significant crustal thickening far inland near the middle of the continent? Do we have any insight as to what the cause might have been? I believe the answer is an unqualified yes. In short, the dramatic uplift of the Rockies represents the isostatic adjustment of a wedge of buoyant lower crust that had been bulldozed eastward by the flat-subducting Farallon Plate before it dropped into the deeper mantle. Details of this scenario are described in a paper by Bird (1988), “Formation of the Rocky Mountains.”1
Stated in other words, the Farallon slab, which was subducting horizontally beneath western North America during the latter stages of the Flood (Cretaceous time in the standard chronology), exerted tractions on the base of the overlying granitic crust, dragging some of the warm and ductile lower crust eastward as it went, thus forming a growing wedge of material concentrated at the front of the horizontal section of slab. When the slab finally fell away, it left this relatively narrow zone of buoyant low density material behind, below the existing North American crust, beneath what are now the Rocky Mountains. As the slab sank deeper, its dynamic influence subsided, and this zone with its extra crustal thickness rose isostatically, punching up the mountain belt, much of it along near-vertical faults like those observed today along the Colorado Front Range.
This is one example of how horizontal tectonics can result in significant crustal thickening and subsequent vertical tectonic expression. A simpler and more common example arises from the volcanism commonly associated with subduction. The magmas commonly rise to form plutonic bodies within the overlying continental crust inboard of the subduction zone. This has occurred along much of the western coast of both North and South America, as indicated schematically in the figure below.
The belt of Mesozoic-Tertiary plutonic rocks extending from Alaska to Antarctica, forming much of the mountain belt known as the American Cordillera, was produced by this subduction-caused melting beneath the western margins of these continents. The maps below display the main sites of this plutonic emplacement of new crustal material. Isostatic adjustment in these belts has resulted in the mountains we see there today.
Probably the most spectacular example of horizontal plate motions resulting in dramatic vertical tectonics involves the formation of the Himalayan Mountains and the Tibetan Plateau. Many lies of evidence indicate that more than 1000 km of the Indian subcontinent has subducted beneath southern Asia. Although seismic studies are still seeking to determine the fates of the mantle portions of the two plates involved, it currently appears that the mantle lithosphere from both plates has detached from the crustal portions and is currently sinking below Tibet, continuing to drive convergent motion as indicated by present-day GPS measurements.
From seismology it is clear that the crustal thickness is between 60 and 75 km in most of this region, which is twice the typical continental value. There are many lines of evidence indicating this increase in crustal thickness and the resulting uplift of the Himalaya and Tibetan Plateau are relatively recent, with the crustal thickening having begun no earlier than Eocene. The uplift of the Himalayas and the Tibetan Plateau must be the isostatic response to dramatic crustal thickening that seismology indicates is genuine. In terms of the Flood, this thickening seems to have taken place near end of the cataclysm. Horizontal tectonics that thrust the Indian plate beneath Asia for about a thousand kilometers appears to be the only plausible way to account for this doubling of the crustal thickness in this region.
Up to this point I have focused on vertical tectonics associated with uplift. But horizontal tectonics can also lead to downwarping of the continental surface when the negative buoyancy of an oceanic plate is coupled mechanically in sufficient degree to a continental plate. This situation can occur when an oceanic slab subducts horizontally beneath a continental plate. Such downwarping was one of the several consequences of the Cretaceous near-horizontal subduction of the Farallon Plate beneath western North America. The resulting depression is known as the Western Interior Seaway, or the North American Cretaceous Seaway, shown in the figure below. It was a relatively shallow sea, but the sediments which filled it preserved abundant marine life, including predatory marine reptiles known as mosasaurs up to 18 meters in length.
To this point we have examined important aspects of vertical tectonics that seem to require horizontal tectonics to account for them. What about the reasons the advocate for vertical tectonics gives for his own rejection of horizontal tectonics in general and plate tectonics in particular? These include 1) the character of the sediments found in trenches 2) the complexity of the magnetization patterns found in ocean bottom basalts, 3) the small amounts of magma beneath the mid-ocean ridges, 4) no subduction zones associated with the Ural and Transantarctic Mountains, and 5) various reasons to him that subduction seems unlikely. These in my view are all trivial objections, especially given the many and powerful lines of evidence that both seafloor spreading and subduction are real. I discuss most of these issues in my answers to questions 3-17 that deal mostly with the topic of subduction. In these answers I address, for example, the characteristics of sediments in the Nankai Trench off Japan and the reality of fossil subduction zones. I also address the reason for the small amounts of magma beneath mid-ocean ridges by pointing out that mid-ocean ridges are generally passive features. I discuss the character of the magnetization of seafloor basalt in question 34 below.
Since I have mentioned the Farallon Plate in so many contexts in this response, let me again include the seismic tomography images I included in my answer to question 8 from a recent paper by Sigloch (2008).3 Figure 2 from this paper, reproduced below, shows via seismic tomography the present shape of the Farallon Plate, which has subducted beneath the western coast of North America since the earliest Jurassic and continues to do so today as the modern Juan de Fuca Plate along the coasts of Oregon and Washington. The Farallon Plate today is extremely deformed and contorted, as these tomography images reveal. The paper provides a great deal of discussion on the history of subduction of this plate and what can be learned from its configuration today. The abstract from the paper is as follows:
Eastward subduction of oceanic tectonic plates has shaped the geologic history of western North America over the past 150 million years. The mountain-building and volcanism that brought forth the spectacular landscapes of the West are credited to the vast ancient Farallon plate, which interacted mechanically and chemically with the overlying continent as it plunged back into the mantle. Here, we use finite-frequency travel-time and amplitude measurements of teleseismic P-waves in seven frequency bands to obtain a high-resolution tomographic image to ~1,800 km depth. We discover several large, previously unknown pieces of the plate which show that two distinct stages of whole-mantle subduction are present under North America. The currently active one descends from the Pacific Northwest coast to 1,500 km depth beneath the Great Plains, whereas its stalled predecessor occupies the transition zone and lower mantle beneath the eastern half of the continent. We argue that the separation between them is linked to the Laramide era 70–50 Myr ago, a time of unusual volcanism and mountain-building far inland generally explained by an episode of extremely flat subduction.
This seismic tomography image to me is strong documentation of the reality of the subduction process and of the profound impact that the subduction of the Farallon Plate had on the geological history of the western North America.
Next, what about the topography associated with abyssal hills, which was offered as a noteworthy example of vertical tectonics? Below is a high resolution sonar image of these low-amplitude, nearly parallel, rill-like features in a portion in the southeastern Pacific Ocean.
These features which are aligned with the nearby spreading ridge are almost certainly product of the seafloor spreading process at the ridge, possibly as magma is emplaced there in quasi-periodic episodes. Once formed, they remain part of the seafloor surface texture until they are buried in sediment. The varying height across them likely has to do with the manner in which the slab-like volume of injected magma cools, with the slowest cooling zone in the center having the highest final elevation.
Finally, what about overthrust faults and strike-slip faults such as the San Andreas which seem to imply larger horizontal motions than vertical tectonics processes can provide? Yes, there is good field evidence that overthrusts like the Keystone Thrust west of Las Vegas, Nevada, pictured below, represent significant horizontal displacements of large sheets of rock of at least several tens of kilometers. Most of the displacement occurred in the late Cretaceous when the Farallon slab was subducting horizontally beneath western North America. However, in my opinion, the force responsible for motion of the upper block on this fault must have been gravity from elevated topography to the west, with this dynamic topography arising from the rapidly moving Farallon slab below.
In regard to the San Andreas Fault, observations indicate at least 500 km (300 miles) of displacement along it since it formed. Such distance corresponds, for example, to the distance of Cabo San Lucas lies from the point on the Mexican coast where Baja California broke away. How much clearer evidence should an open minded person require to be persuaded that large-scale horizontal tectonics motions are indeed real?
-
Peter Bird, “Formation of the Rocky Mountains, Western United States: A Continuum Computer Model,” Science 239, 1501-1507, 1988. ↩︎
-
J. D. Winter, An Introduction to Igneous and Metamorphic Petrology, Prentice Hall, 2001. ↩︎
-
K. Sigloch, N. McQuarrie, and G. Nolet, “Two-stage subduction history under North America inferred from multiple-frequency tomography,” Nature Geosciences, 1, 458-462, 2008 ↩︎
-
J. A. Goff et al., “The contributions of abyssal hill morphology and noise to altimetric gravity fabric,” Oceanography 17, 24-37, 2004. Online link ↩︎