CPT Q. 013: Why are some Benioff zones nearly horizontal?
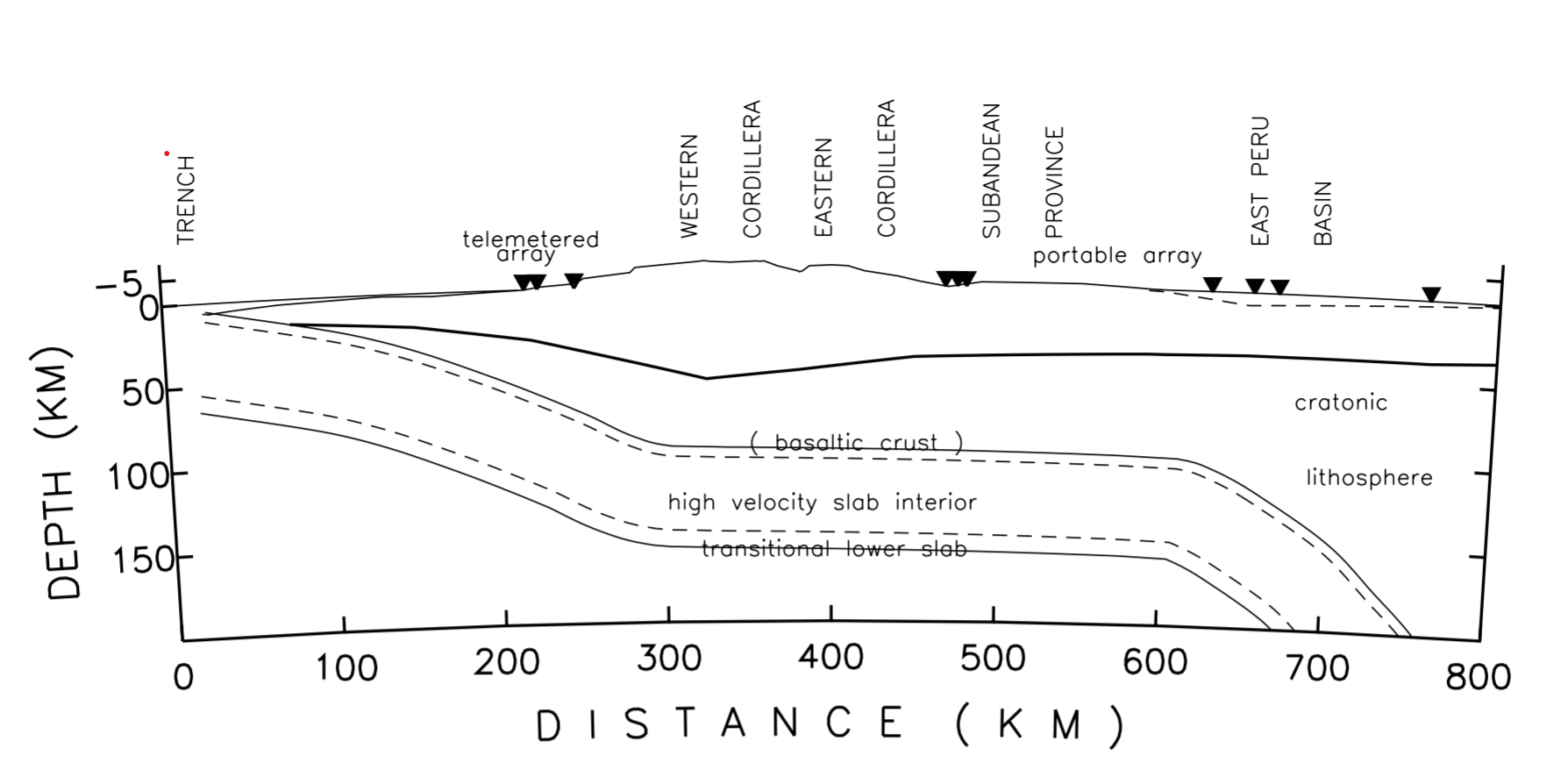
Q. 13. Why are some Benioff zones nearly horizontal when subducting plates should be expected to plunge downward at a finite angle?
Response: This question is motivated by the sentence from the abstract of the 2004 paper by Booker et al., “Low electrical resistivity associated with plunging of the Nazca flat slab beneath Argentina,”1, that reads:
“But between 28° and 33° S the subducted Nazca Plate appears to be anomalously buoyant, as it levels out at about 100 km depth and extends nearly horizontally under the continent.”
The full abstract reads,
Beneath much of the Andes, oceanic lithosphere descends eastward into the mantle at an angle of about 30°. A partially molten region is thought to form in a wedge between this descending slab and the overlying continental lithosphere as volatiles given off by the slab lower the melting temperature of mantle material. This wedge is the ultimate source for magma erupted at the active volcanoes that characterize the Andean margin. But between 28° and 33° S the subducted Nazca Plate appears to be anomalously buoyant, as it levels out at about 100 km depth and extends nearly horizontally under the continent. Above this ‘flat slab’, volcanic activity in the main Andean Cordillera terminated about 9 million years ago as the flattening slab presumably squeezed out the mantle wedge. But it is unknown where slab volatiles go once this happens, and why the flat slab finally rolls over to descend steeply into the mantle 600 km further eastward. Here we present results from a magnetotelluric profile in central Argentina, from which we infer enhanced electrical conductivity along the eastern side of the plunging slab, indicative of the presence of partial melt. This conductivity structure may imply that partial melting occurs to at least 250 km and perhaps to more than 400 km depth, or that melt is supplied from the 410 km discontinuity, consistent with the transition-zone ‘water-filter’ model of Bercovici and Karato.
Note that beyond that flat zone the slab “finally rolls over to descend steeply into the mantle.”
Flat subduction has been shown by seismic studies to be occurring today in several places in the world, two separate segments in fact beneath South America including the one just described. A seismic investigation of the northern segment beneath Peru is contained in a 1992 Virginia Tech master’s thesis by E. O. Norabuena entitled “Velocity structure of the subducting Nazca Plate beneath central Peru as inferred from travel time anomalies”2.The findings of this study are summarized in the figure below:
Note that like the similar zone beneath Chili and Argentina, the slab after moving nearly horizontally, in this case for only about 300 km, then plunges downward at a steep angle. This thesis also includes a map showing the tectonic setting, shown below.
There is also a strong case that the Farallon Plate that subducted beneath the western coast of North America. This was first proposed more than 20 years ago by P. Bird in “Formation of the Rocky Mountains…”3 The abstract of this paper is as follows:
One hypothesis for the information of the Rocky Mountain structures in late Cretaceous through Eocene time is that plate of oceanic lithosphere was underthrust horizontally along the base of the North American lithosphere. The horizontal components of the motion of this plate are known from paleomagnetism, and the edge of the region of flat slab can estimated from reconstructed patterns of volcanism. New techniques of finite-element modeling allow prediction of the thermal and mechanical effects of horizontal subduction on the North American Plate. A model that has a realistic temperature-dependent rheology and a simple plane-layered initial condition is used to compute the consequences of horizontal underthrusting in the time interval 75 million to 30 million years before present. Successful prediction of this model include (i) the location, amount, and direction of horizontal shortening that has been inferred from Laramide structures; (ii) massive transport of lower crust from southwest to northeast; (iii) the location and timing of the subsequent extension in metamorphic core complexes and the Rio Grande rift; and (iv) the total area eventually involved in Basin-and-Range style extension.
In a broad sense, this model has predicted the belt of Laramide structures, the transport of crust from the coastal region to the continental interior, the subsequent extension in metamorphic core complexes and the Rio Grande rift, and the geographic region of late Tertiary Basin-and-Range extension. Its principal defects are that (i) many events are predicted about 5 million to 10 million years too late and (ii) the wave of crustal thickening does not travel far enough to the east. Reasonable modifications to the oceanic plate kinematics and rheologies that were assumed may correct these defects.
The correspondence of model predictions to actual geology is already sufficiently close to show that the hypothesis that horizontal subduction caused the Laramide orogeny is probably correct. The Rocky Mountain thrust and reverse faults formed in an environment of east-west to northeast-southwest compressive stress that was caused by the viscous coupling between the oceanic plate and the base of the North American crust. Nonuniform crustal thickening by simple-shear transport also caused relative uplifts; therefore, this model is consistent with both of the range-forming mechanisms that have been inferred. A new proposal that arises from this simulation is that horizontal subduction also caused the subsequent extensional Basin-and-Range taphrogeny by stripping away the mantle lithosphere so that the crust was exposed to hot asthenosphere after the oceanic slab dropped away.
This landmark paper has been widely referenced in subsequent work on the geology of the western United States. The inference of a period of flat subduction by the Farallon Plate beneath western North America is prominent in the much more recent paper by Sigloch, McQuarrie, and Nolet (previously mentioned in my response above to question 8 that presents the 3D seismic tomography image of the strongly contorted Farallon Plate. The author’s interpretation of the 3D tomographic image in terms of the subduction history of the Farallon Plate is provided in the figure below, reproduced from their paper:
Of course, the interpretation in this article as well as in the previous one is in terms of the uniformitarian time scale, which is to be rejected in regard to as far as absolute dates are concerned. In summary, the case for flat subduction of slabs is compelling, not only in the present but also in the past. Numerical models show that it is mechanically plausible. The main driving force for moving the slab is the slab pull arising from the negative buoyancy of the cold dense material that comprises the slab. These conclusions apply equally for UPT and CPT.
-
Ibid, Nature 429, 399-403, 2004. ↩︎
-
P. Bird, “Formation of the Rocky Mountains, Western United States: A Continuum Computer Model,” Science 239, 1501-1507. Some links to find it: author’s site abstract, author’s Google Scholar profile, and author’s listed link on last). ↩︎