CPT Q. 004: Are sediments, volcanoes, and plateaus actually scraped off subducting plates at trenches?
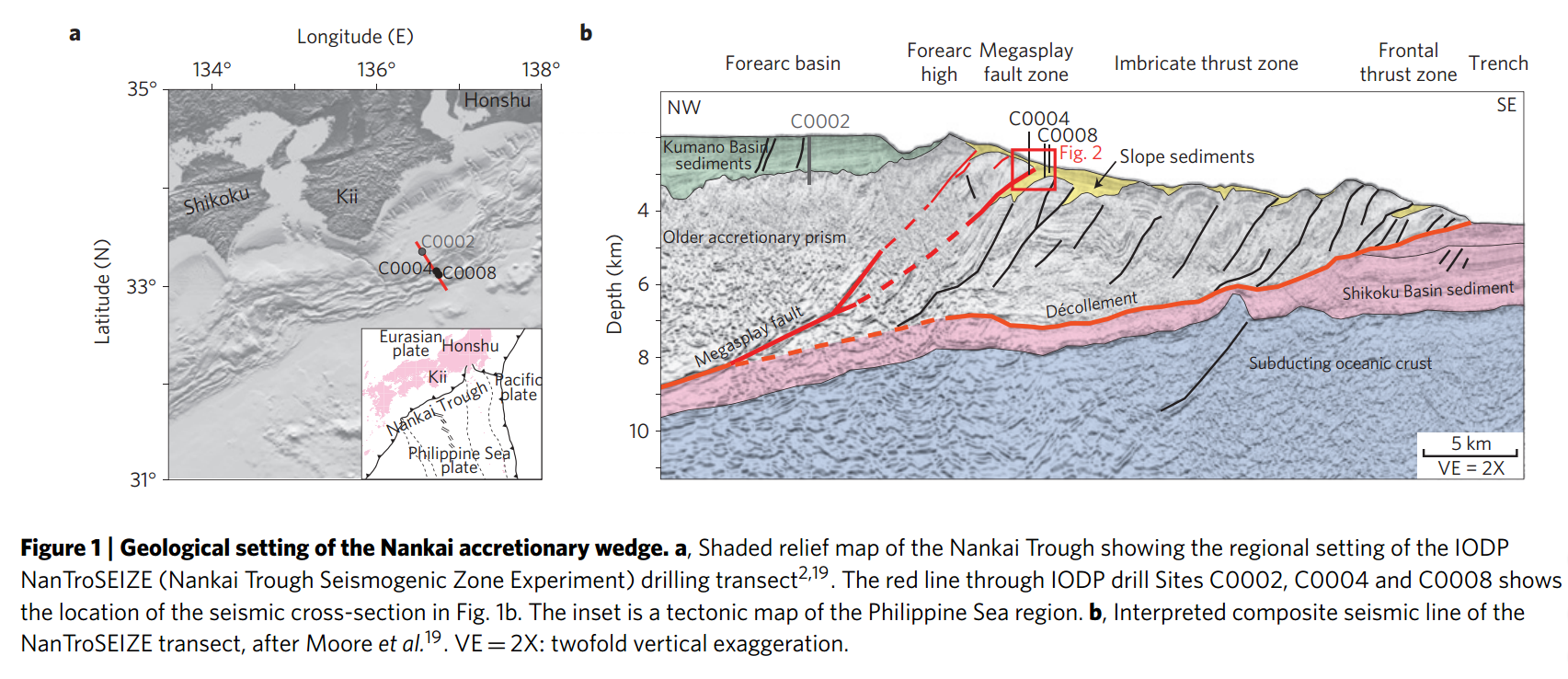
Q. 4. What evidence supports the idea that sediments, volcanoes, and plateaus have been scraped off subducting plates at trenches?
Response: There are thousands of papers documenting the reality of accretionary wedges at subduction zones in the standard literature. Let me pick just one published 16 August 2009 in Nature Geoscience by M. Strasser et al. entitled: “Origin and evolution of a splay fault in the Nankai accretionary wedge” (available here [ed. link updated from]). This paper deals with the faulting history within the accretionary wedge that lies within the Nankai Trough, where the Philippine Sea plate is actively subducting below southwest Japan and repeatedly producing destructive earthquakes and tsunamis. Figure 1 from this paper is reproduced below. Panel a, which is a shaded relief map of the region, shows the corrugated upper surface of the wedge, spanned by the red line segment, between the relatively smooth sediment covered top of the Philippine Plate to the southeast and the smooth floor of the Kumano Basin to the northwest. Panel b shows the result of a seismic transect along this line segment. This figure shows a portion of the Philippine Plate that is actively subducting (as indicated by 13 major earthquakes during the last 1300 years, 11 of which are estimated to have been greater than magnitude 8.0) beneath a thick wedge of accreted sediments which are highly contorted and sliced by numerous internal faults, including the megasplay fault shown in red on the left in panel b. Some of these earthquakes generated tsunamis greater than 25 m high. (For more details, see here) It is believed that slip on the megasplay fault during the large earthquakes is responsible for the large-amplitude tsunamis. Sediments recovered in the three drill cores that were an important part of this study suggest that splay fault activity has varied strongly through time, with alternating high-activity periods during which splay-fault thrusting accommodated a large part of the plate convergence. One possible cause the authors cite for this variation is roughness changes on the incoming plate (such as the presence ridges or seamounts).
Another example of observational support for the reality of subducted sediment and seafloor volcanic cones accumulating in subduction zones is the classic example of the Franciscan terrane along some 650 km of the California coast mostly north of San Francisco.1,2,3,4 These Franciscan rocks represent the trench complex of a Mesozoic, east-dipping subduction zone beneath the western coast of North America. During that time period the Farallon Plate was being subducted beneath the overriding North American Plate. Simultaneous with the formation of these subduction zone rocks was the development of a volcanic arc system that produced the Sierra Nevada batholith. The rock units in today’s Franciscan terrane are derived from sediments deposited in an accretionary wedge as well as from slices of the underlying oceanic crust and mantle. Rock ages range from uppermost Jurassic through Cretaceous. The sediments of the accretionary wedge are medium- to fine-grained detrital rocks (graywackes, micrograywackes, and dark shales) derived from the Sierran volcanic-plutonic arc. They were deposited into the trench as turbidites. Not only were substantial volumes of these sediments were subducted atop the downgoing oceanic plate, in this subduction process they were also metamorphosed to high metamorphic grade!
How deeply were these sediments subducted to reach this degree of metamorphism? The petrology and geochemistry of the minerals in the metamorphosed graywackes and off-scraped basalts yield the answer. For example, the albite present in the original sedimentary and igneous rock has been converted into jadeitic pyroxene plus quartz. This reaction can only occur in a high pressure, low temperature environment. Laboratory experiments indicate temperatures of about 200°C and pressures of about 0.6-0.7 GPa. This corresponds to depths of about 20 to 30 km in a subduction zone. Another metamorphic mineral that constrains the pressure-temperature conditions of the metamorphism is glaucophane. Glaucophane (meaning ‘blue appearing’ in Greek) belongs to the amphibole family of minerals and has the chemical formula Na2(Mg,Fe)3Al2Si8O22(OH)2. Its name comes from its typical blue color. Rocks containing significant amounts of glaucophane are generally referred to as blueschists. The mix of metamorphic rock types commonly associated with blueschist is referred to as the ‘blueschist facies.’ Blueschist facies rocks generally form under pressures of greater than 0.6 GPa, equivalent to depth of burial in excess of 15-18 km, and at temperatures of between 200 to 500 °C. This is a ’low temperature, high pressure’ prograde metamorphic path and is also known as the Franciscan facies series, after this Franciscan complex of California where these rocks are so magnificently exposed. Similar blueschists in similar tectonic settings also occur in Greece, Turkey, Japan, New Zealand and New Caledonia.
A major issue associated with the occurrence of these blueschist facies rocks, however, is how they manage return to near the earth’s surface after reaching the pressure conditions needed for their formation. Minerals such as jadeite and glaucophane revert to lower pressure phases as they rise to the surface if their temperatures are much above 200°C. The standard geological community freely acknowledges this is an unsolved problem and has recognized this reality for the past forty years. Whatever the process, they acknowledge that it must be rapid on order to preserve the high-pressure, low temperature minerals. Most acknowledge that the positive buoyancy of the metamorphosed rocks must be a key factor. Jadeitic pyroxene is less dense and more viscous than olivine that occurs in the mantle. This difference in buoyancy allows the subducted crustal rock to rise. They also marvel at the fact that many of the Franciscan tectonic blocks, some at least 10 km in lateral dimension, have been returned to the surface relatively intact (that is, stratigraphically coherent) although locally very contorted.
One primary difficulty for the mainstream community is how quickly these high-pressure, low-temperature metamorphic minerals must return to the surface in order to escape conductive heating at the depths where the metamorphism takes place. The key issue here is the time scale, and in the uniformitarian framework, the typical time span inferred between subduction and exhumation implies too much heating at depth. This difficulty, of course, disappears in the CPT framework because the subduction and exhumation velocities are orders of magnitude higher and the time scale is orders of magnitude shorter.
A second major issue is the volume of the sediments that are able to be entrained by the downgoing oceanic plate. The higher the speed of downgoing plate, the higher are the shear stresses that act on potentially entrained sediments. The greater these shear stresses are, the thicker in general will be the layer of subducted sediment. Furthermore, the larger the volume of entrained sediment carried to depth, the more readily and faster that volume—because of its buoyancy— will be able to rise again to the surface. Whereas in UPT the subduction and exhumation of huge volumes of blueschist facies rocks observed in the Franciscan complex are nearly impossible to account for, within the CPT framework they are to be expected. For these reasons, the blueschist facies terranes from many places in the world represent powerful support for the reality of CPT.
Why have I devoted so much space to discussion of the Franciscan rocks? First, this complex represents a place on earth where one can actually observe first-hand a sequence of rocks some 100 km wide that accumulated in an ocean trench as an accretionary wedge by subduction processes in the past. As such, these rocks testify powerfully that past subduction is genuine and unambiguous. Second, these rocks reveal clearly that buoyant sediments can be and have been subducted to significant depths, been metamorphosed, and then, because of their buoyancy, rapidly returned to the surface. Third, these rocks indicate that processes different in some way from those acting presently were involved. The simplest way to account for the large volumes of sediment involved as well as the rapidity they returned to the surface is dramatically higher plate speeds. In other words, these rocks testify strongly that past subduction was distinctly different in some ways from what is currently occurring today. By way of summary, these field examples of subduction, while understandable in the framework of conventional plate tectonics, fit more consistently within the CPT framework in that CPT can more readily account for such features as blueschist facies rocks in older accretionary complexes.
Finally, in regard to whether or not oceanic plateaus have been scraped off by subduction, there are numerous papers that document that a significant portion of western North America is the result of what is sometimes referred to as ‘collage tectonics’. Indeed, the recognition that many modern and ancient continental margins are comprised of diverse crustal fragments, each with a distinctive stratigraphy and separated by tectonic contacts, initially occurred for the Pacific coast part of North American in the 1970’s. Over 200 distinct terranes have been now been identified in this portion of North America, which includes most of Alaska, much of British Columbia, and major portions of California, Oregon, and Washington. This region is now understood to be a collage of oceanic plateaus, oceanic volcanic arcs, and continental fragments, brought together brought together over large distances (in many cases more than a thousand km) by plate motions and plate convergence. Other continental regions including eastern Siberia, much of China, south-central and southwestern Asia, central Europe, eastern Australia, and southeastern New England, are also now recognized to be the amalgamation of diverse crustal fragments that have been brought together by plate convergence and subduction. Because this literature is so vast, I simply provide below as an example a summary description of the collage tectonics aspects of British Columbia written as a student assignment in 2005 and posted here [ed. updated original link]. I have shortened it slightly and omitted the list of references:
TECTONIC EVOLUTION OF NORTHERN BRITISH COLUMBIA by Chad Seigel
Introduction: Northern British Columbia is mountainous area composed of various faults and orogenic belts which are part of a larger geographic area known as the Canadian Cordillera. This area is composed of ancient cratonic basement, and a collage of terranes which later accreted to this basement as shown in the figures below. This report will focus on a transect cut through northern British Columbia from east to west, starting on the BC/Alberta border, running along the 60th parallel and ending on the Alaskan coast. Although much more complex, the five morphological belts and the ancient cratonic basement which are located along this transect will be briefly summarized in terms of the terranes of which each belt is composed and tectonic origin and time of accretion.
Ancient Continental Margin: The ancient continental margin of North America was formed during late Proterozoic when continental rifting tore the former supercontinent Rodinia apart creating ancestral North America (Laurentia). This resulted in the formation of a passive continental margin much like the present day east coast of North America which persisted until middle Devonian. During this time a thick miogeoclinal deposit of sediment which was eroded from the Canadian Shield to the east was deposited on the continental shelf of the proto Pacific Ocean known as Panthalassa. This supracrustal wedge increases in thickness from east to west where it is approximately 5 km thick at the edge of the Foreland Belt.
Foreland Belt: Moving from east to west along the transect the Foreland Belt is encountered. Although this belt is the next in the sequence of orogenic belts it was the last to form. During the break up of Pangea during the Jurassic the North American continent began to move westward like a giant bulldozer accreting terranes which lay just off the coast of the continent. In doing so these terranes were squeezed in a giant vice between the subducting oceanic lithosphere off the coast of North America, and the wedge shaped North American craton. These terranes were squeezed upwards and downwards which resulted in the detachment of the thick miogeoclinal sequences deposited during the late Proterozoic and Paleozoic from the cratonic basement. These sequences were upthrust onto the edge of the North American craton forming the Foreland Belt.
Omenica Belt: Next along the transect is the Omenica Belt. The Omenica Belt is the region of overlap between the Intermontaine Belt to the west and the Foreland Belt to the east. During the late Devonian, it is thought that an oceanic trench was created at the continent ocean boundary caused by the enormous weight of the old dense oceanic crust combined with the weight of sediments deposited on the continental shelf. Stratigraphic and U-Pb age constraints indicate that this episode of magmatism was Devonian-Mississippian and has been interpreted as subduction related magmatism at a convergent margin. Today, nowhere on Earth is there evidence of subduction being initiated, and the cause of change from a passive, intra-plate margin to a convergent, inter-plate margin is uncertain. This newly formed convergent boundary created magmatic island arcs which formed on the edge of the North American craton not far from the continental margin. These arcs are the Slide Mountain, Cassiar, and Yukon-Tanana Terranes which make up the Omenica Belt. These two arcs with their associated back arc basins have gone through numerous metamorphic events throughout their history, and today represent the once deeply buried roots of this ancient magmatic arc assemblage.
Intermontaine Belt: The Intermontaine Belt is composed of the Cache Creek, Nisling, and Stikine terranes. The Cache Creek and Nisling terranes were formed in the western Pacific during the Permian to Middle Triassic. Sedimentary rocks of the Cache Creek Terrane contain a particular assemblage of fossils that are found in Asia and not in ancient North American rocks. This suggests that the Cache Creek Terrane likely originated far from North America and may have existed on the other side of the Pacific Ocean. The Stikine Terrane is a Carboniferous to Early Jurassic island arc which was formed in the east Pacific. The Stikine Terrane is believed to have evolved in the east Pacific of the Northern Hemisphere and moved northward to dock with ancestral North America sometime during the Middle Jurassic. During the Early to Middle Jurassic the two terranes joined to form the Intermontaine Belt. After this time arc related magmatic activity continued into the Tertiary. Late Triassic through Tertiary plutons intrude structurally imbricated Stikine and Cache Creek terranes in the Atlin-Bennett Lake area of northern British Columbia.
Coast Belt: The Coast Belt is the suture zone between the Intermontaine Belt and the Insular Belt and is composed of plutonic and metamorphic rock. During the mid Cretaceous, the exotic Insular superterrane collided with North America, further deforming the Intermontaine terranes. The deformation compressed the upper crust of the Nisling and Stikine terranes by more than 160 km, approximately the width of the Coast Belt.
Insular Belt: Westernmost is the Insular Belt which is composed of the Wrangel, Alexander, Chugach, and Yukatat terranes. The Wrangel and Alexander terranes are island arcs having formed in the Pacific during the Devonian. These arcs amalgamated during the Carboniferous and collided with the continent during the Cretaceous. It is uncertain exactly when the Insular Islands finally arrived on North American shores. Some evidence suggests they persisted as an offshore volcanic chain for some time, much like the islands of Japan do today. In any event, the final collision between the islands and the continent did not occur until mid-Cretaceous time. The Chugach and Yukatat terranes are composed of sedimentary rock of Tertiary age. Products of erosion were deposited in Pacific Ocean floor and carried northward on the oceanic plate to be accreted as major components of Chugach and younger accretionary terranes southern Alaska.
Summary: The collage of terranes and faults that make up the continental crust of northern British Columbia are extremely complex and have been simplified here for clarity. Much is currently known about the complex geology of this area and many questions still remain unanswered. With new theories and geoscience techniques more questions and answers will be brought to light, once again changing our understanding of the tectonic evolution of northern British Columbia. (End of article)
Although these striking evidences for long distance transport and assembly of these terranes in a collage-like manner is accepted within the UPT framework by the mainstream earth sciences community, it is a major stretch to imagine that the slow and gradual plate motions we observe today could have achieved such incredible tectonic feats. It is dramatically more reasonable, however, that these sorts of amalgamations could and did take place if the plate velocities were orders of magnitude higher than they are today during a brief but intense cataclysm.
-
Ernst, W. G., “Tectonic contact between the Franciscan mélange and the Great Valley Sequence—crustal expression of a late Mesozoic Benioff zone,” J. Geophys. Res., 75, 886-901, 1970. ↩︎
-
Ernst, W. G., “Petrologic reconnaissance of Franciscan metagraywackes from the Diablo Range, central California Coast Ranges,” J. Petrol., 12, 413-437, 1971. ↩︎
-
Ernst, W. G., “Metamorphism of Franciscan tectonostratigraphic assemblage, Pacheco Pass area, east-central Diablo Range, California Coast Range,” Geol. Soc. America Bull., 105, 618-636, 1993. ↩︎
-
Tembayashi, M., S. Maruyama, and J. G. Liou, “Thermobaric structure of the Franciscan Complex in the Pacheco Pass Region, Diablo Range, California,” J. Geol., 104, 617-636, 1996. ↩︎